In this article, we have covered 10 mind-blowing facts about space and Universe that will leave you in awe.
The universe is a vast and mysterious expanse that has fascinated humans for centuries. Its sheer size, complexity, and the wonders it holds never fail to inspire awe and wonder.
From mind-boggling celestial phenomena to mind-blowing discoveries, the universe presents us with an endless array of fascinating facts that challenge our understanding of the cosmos.
In this exploration of mind-blowing facts about the space and universe, we will delve into the realms of astronomy, astrophysics, and cosmology to uncover astonishing phenomena, mind-expanding concepts, and awe-inspiring wonders that will leave you contemplating the vastness and beauty of our universe.
Brace yourself for a journey that will challenge your imagination and ignite a sense of wonder about the mysteries that lie beyond our planet.
Get ready to be amazed by the mind-blowing facts about the space and universe that will expand your horizons and leave you in awe of the cosmic wonders that surround us.
10 Mind-Blowing Facts About Space and Universe
1. Vastness of the Universe
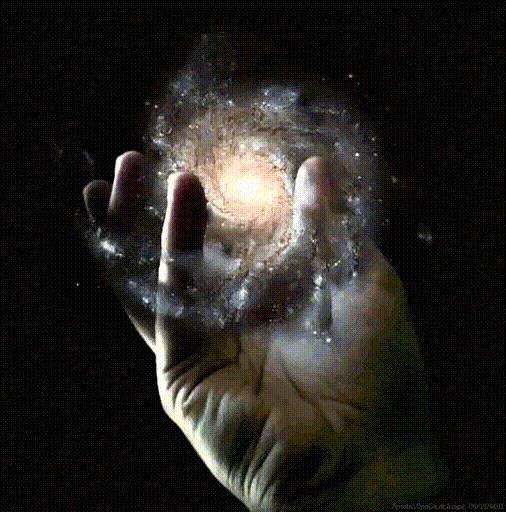
The universe is unimaginably vast, containing billions of galaxies, each with billions of stars. The sheer scale of the cosmos is awe-inspiring, with our own Milky Way galaxy-spanning approximately 100,000 light-years or about 30 kpc.
In the vastness of the cosmic space, you are like a grain of sand. #SadhguruQuotes pic.twitter.com/cxExYp570H
— Sadhguru (@SadhguruJV) November 2, 2017
The vastness of the universe refers to its immense size and the abundance of celestial objects within it. The universe is a vast expanse that encompasses everything we know, from galaxies and stars to planets and cosmic structures.
The universe is estimated to be around 13.8 billion years old since the Big Bang, which is believed to have initiated its expansion. Its size is difficult to comprehend, but the observable universe alone is estimated to have a diameter of about 93 billion light-years. This means that even if we travel with the speed of light, approximately 186,282 miles per second (299,792 kilometers per second), would take 93 billion years to traverse its entire span.
Within the universe, galaxies are the building blocks. They are enormous collections of stars, gas, dust, and dark matter. The Milky Way, our home galaxy, contains billions of stars, and it is just one of the estimated 100-200 billion galaxies in the observable universe. Each galaxy has its own unique structure and characteristics, further amplifying the vastness of the cosmos.
Stars themselves are captivating objects. Ranging in size, temperature, and brightness, they fill galaxies with their light and energy. Our Sun is a relatively average star, but there are stars much larger and brighter than it scattered throughout the universe. The number of stars in the universe is staggering, estimated to be in the hundreds of billions of trillions (10^21) or even more.
The discovery of exoplanets has revealed the existence of numerous planets beyond our solar system. There could be billions of potentially habitable planets throughout the Milky Way alone, and considering the vast number of galaxies, the total number of planets in the universe is mind-boggling.
Moreover, the universe is constantly expanding. The galaxies are moving away from each other, driven by the force of cosmic expansion. This expansion adds to the unfathomable vastness of the cosmos, as it suggests that there are regions of space beyond what we can currently observe.
Overall, the vastness of the universe is a testament to its awe-inspiring scale, beauty, and potential for exploration and discovery. It challenges our understanding and encourages us to continue exploring and unraveling its mysteries.
2. Cosmic Web
The structure of the universe is organized into a vast network of filaments and voids known as the cosmic web. These filaments are composed of galaxies and other cosmic matter, stretching across billions of light-years.
The cosmic web is a term used to describe the large-scale structure of the universe. It refers to the intricate network of galaxies, galaxy clusters, filaments, and voids that make up the cosmic structure on a vast scale.
The cosmic web forms as a result of the gravitational interactions between matter in the early universe. After the Big Bang, the universe started expanding and matter began to clump together under the influence of gravity. Over billions of years, these clumps of matter grew larger and merged, forming massive structures known as galaxy clusters. These clusters are interconnected by long, thread-like structures called filaments.
The filaments of the cosmic web act as cosmic highways, guiding the flow of matter and galaxies across the universe. They can stretch for hundreds of millions of light-years and serve as pathways along which galaxies are distributed. The filaments intersect and form nodes, where multiple filaments converge. These nodes are often the locations of large galaxy clusters.
In between the filaments, there are vast regions known as voids. These voids are relatively empty spaces with a lower density of matter compared to the filaments and clusters. They can be tens of millions of light-years in diameter.
The cosmic web is a remarkable representation of the large-scale structure of the universe. It provides insights into the formation and distribution of galaxies on a cosmic scale. Scientists study the cosmic web to understand the dynamics of the universe, the evolution of structures, and the underlying forces shaping its structure.
Mapping and understanding the cosmic web are challenging tasks. However, observations from large-scale surveys and computer simulations help us visualize and study this intricate cosmic architecture, shedding light on the vast and complex structure of our universe.
3. Dark Energy and Dark Matter
The majority of the universe is composed of mysterious dark energy and dark matter. Dark energy is believed to be responsible for the accelerating expansion of the universe, while dark matter’s gravitational effects bind galaxies and other cosmic structures together.
Dark energy and dark matter are two mysterious components of the universe that play significant roles in its structure and expansion. While they are still not fully understood, scientists have gathered evidence for their existence based on observations and their effects on the cosmos.
Dark Matter
Dark matter refers to an invisible and elusive form of matter that does not interact with light or other forms of electromagnetic radiation. It does not emit, absorb, or reflect light, making it difficult to directly detect. However, its presence is inferred by its gravitational effects on visible matter and the way it influences the motion of galaxies and galaxy clusters.
The gravitational pull of dark matter is believed to be responsible for holding galaxies together and ensuring they don’t fly apart due to their high rotational speeds. It also plays a crucial role in the large-scale structure of the universe, acting as a scaffolding around which galaxies and clusters form.
Despite its name, dark matter is thought to be more abundant in the universe than visible matter. It is estimated to make up around 85% of the total matter content of the universe. However, its exact nature and composition remain unknown. Various theories propose that dark matter could consist of undiscovered subatomic particles that interact only weakly with normal matter.
Dark Energy
Dark energy, on the other hand, is a theoretical form of energy that is believed to permeate all of space and is associated with the accelerated expansion of the universe. Unlike dark matter, dark energy does not cluster or clump together but appears to be evenly distributed throughout the universe.
The discovery of dark energy is relatively recent, dating back to the late 1990s when astronomers studying distant supernovae observed that the universe’s expansion was accelerating, contrary to expectations. Dark energy is believed to counteract the gravitational pull of matter, driving the accelerated expansion of the universe.
The nature of dark energy remains highly enigmatic. One possibility is that it is related to the energy inherent in space itself, often referred to as the cosmological constant. Other theories propose the existence of dynamic fields or modifications to Einstein’s theory of general relativity to explain the observed accelerated expansion.
While dark energy and dark matter are distinct phenomena, they both contribute to our understanding of the universe’s composition and behavior. Their existence challenges our current understanding of fundamental physics and continues to be an active area of research, with scientists working to unravel their mysteries and shed light on the nature of these elusive components.
4. Cosmic Microwave Background Radiation
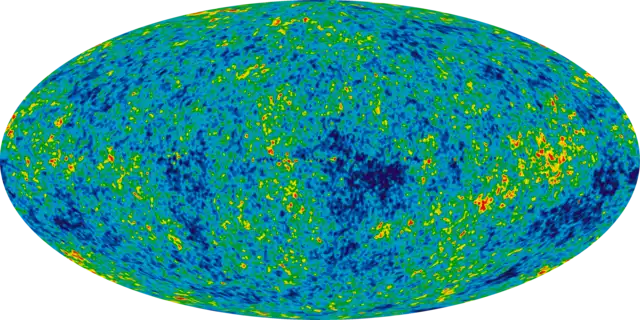
The cosmic microwave background radiation is a faint glow present in all directions of the universe. It is considered the afterglow of the Big Bang and provides crucial evidence supporting the Big Bang theory.
The cosmic microwave background (CMB) radiation is a fundamental aspect of the universe that provides valuable insights into its early stages and subsequent evolution. It is a faint, uniform glow of microwave radiation that permeates the entire observable universe.
The CMB radiation is considered a remnant of the early universe, specifically from a time known as the “epoch of recombination.” Around 380,000 years after the Big Bang, the universe had cooled enough for protons and electrons to combine and form neutral hydrogen atoms.
This process, known as recombination, marked the transition from an opaque plasma to a transparent universe.
Prior to recombination, photons were continuously scattered by the free electrons present in the hot plasma, making the universe opaque. However, once recombination occurred, the photons were able to travel freely without being frequently scattered. These photons have been traveling through space ever since and form the CMB radiation we observe today.
The CMB radiation has several remarkable properties:
Uniformity
The CMB radiation appears remarkably uniform in all directions. It has been measured to have an almost precisely uniform temperature of around 2.7 Kelvin (-270.45 degrees Celsius or -454.81 degrees Fahrenheit) throughout the sky, with only tiny variations at the level of a few parts in a million.
Blackbody Spectrum
The CMB radiation exhibits a blackbody spectrum, which corresponds to the radiation emitted by an object at a specific temperature.
This spectrum provides strong evidence for the Big Bang theory and the early hot and dense state of the universe.
Anisotropies
While the CMB radiation is predominantly uniform, it also contains subtle temperature fluctuations, known as anisotropies.
These variations, mapped with increasing precision by instruments like the Planck satellite, provide valuable information about the density fluctuations in the early universe, which eventually led to the formation of galaxies and large-scale structures.
The precise measurement and analysis of the CMB radiation have been instrumental in our understanding of cosmology, the composition of the universe, and the process of cosmic evolution.
The observed properties of the CMB radiation strongly support the current cosmological model known as the Lambda-CDM model, which describes the universe as being dominated by dark energy and dark matter.
Studying the CMB radiation allows cosmologists to investigate phenomena such as the inflationary epoch, the formation of large-scale structures, and the age, geometry, and composition of the universe. Ongoing and future missions continue to refine our understanding of CMB radiation, enabling us to explore the universe’s origins and unravel its mysteries.
5. Black Holes
Black holes are regions of space where gravity is so strong that nothing, not even light, can escape. They form from the remnants of massive stars and have immense gravitational forces that distort spacetime.
Black holes are fascinating and mysterious cosmic objects that possess such strong gravitational forces that nothing, not even light, can escape their gravitational pull within a certain boundary known as the event horizon. They are formed from the remnants of massive stars that have undergone gravitational collapse.
When a massive star exhausts its nuclear fuel, it can no longer resist the inward pull of gravity. The star’s core collapses under its own gravitational force, causing an immense concentration of mass within a small volume.
If the mass of the core exceeds a critical threshold, known as the Chandrasekhar limit, the collapse continues until a singularity is formed—a point of infinite density at the center. This singularity is surrounded by the event horizon, which marks the boundary beyond which nothing can escape.
Intriguing properties of the Black Holes:
Singularity
At the core of a black hole lies a singularity—a point of infinite density where the laws of physics, as we currently understand them, break down. Our understanding of the singularity is limited, and it is an active area of research in physics.
Event Horizon
The event horizon is the boundary beyond which nothing, including light, can escape the black hole’s gravitational pull. Once an object crosses the event horizon, it is effectively trapped within the black hole and will inevitably reach the singularity.
Spacetime Curvature
Black holes curve the fabric of spacetime around them, creating a gravitational well. The curvature of spacetime becomes more pronounced closer to the black hole, resulting in a one-way flow of matter and energy toward it.
Sizes and Types
Black holes come in different sizes and types. Stellar black holes, formed from the collapse of massive stars, can have masses several times that of our Sun. Supermassive black holes, found at the centers of galaxies, have masses ranging from millions to billions of times that of the Sun.
Effects on Surrounding Matter
As matter spirals into a black hole, it forms an accretion disk—a disk of hot, glowing gas—around it. The immense gravitational forces near the black hole can generate powerful jets of energetic particles that are launched into space.
While black holes themselves do not emit light, they can be indirectly observed through their effects on surrounding matter. Scientists study the behavior of matter falling into black holes, as well as the radiation emitted from the accretion disks and jets, to gather insights into their properties and dynamics.
Black holes are captivating objects that challenge our understanding of gravity, spacetime, and the fundamental laws of physics. They continue to be a subject of extensive research and exploration, with the aim of unraveling their mysteries and deepening our understanding of the universe.
6. Multiverse Theory
The concept of a multiverse suggests that our universe may be just one of many parallel universes. Each universe within the multiverse could have its own set of physical laws and conditions.
The multiverse theory is a speculative concept in theoretical physics that suggests the existence of multiple universes beyond our own. It proposes that our universe may be just one of many universes, each with its own set of physical laws, constants, and initial conditions.
There are different variations of the multiverse theory, and here are a few notable ones:
Many-Worlds Interpretation
According to the quantum mechanics-based Many-Worlds Interpretation, every quantum event creates multiple branching universes, resulting in a multitude of parallel universes. Each universe represents a different outcome or possibility.
Inflationary Multiverse
Inflationary cosmology, which explains the rapid expansion of the early universe, suggests that the inflation process may have led to the formation of multiple bubble-like universes within a larger multiverse. These universes could have different physical properties.
String Theory and Brane Multiverse
String theory, an attempt to unify quantum mechanics and general relativity, proposes the existence of extra dimensions beyond the three spatial dimensions we perceive. In the brane multiverse scenario, our universe is a four-dimensional brane embedded in a higher-dimensional space that contains other branes representing separate universes.
Mathematical Multiverse
The mathematical multiverse idea stems from the concept that mathematical structures and equations exist independently of our physical universe. According to this view, all possible mathematical structures represent distinct universes with their own laws of physics.
It’s important to note that the multiverse theory is still largely speculative, as direct observational evidence for other universes is currently beyond our technological capabilities. However, the concept has gained interest among physicists as a way to explain certain aspects of the cosmos, such as the fine-tuning of physical constants or the observed complexity of our universe.
Further research and theoretical developments are required to explore the validity and implications of the multiverse theory.
It remains an active area of study, with scientists working to refine the mathematical models, investigates potential observational signatures, and explore the philosophical and metaphysical implications of a multiverse.
7. Cosmic Inflation
Cosmic inflation theory proposes that the universe underwent a rapid expansion phase just moments after the Big Bang. This rapid expansion helps explain the universe’s homogeneity and smoothness observed on large scales.
Cosmic inflation is a theory in cosmology that suggests the early universe underwent a rapid and exponential expansion shortly after the Big Bang. It proposes that within a fraction of a second, the universe expanded exponentially, increasing its size by an enormous factor.
The concept of cosmic inflation was first proposed in the early 1980s by physicists Alan Guth and Andrei Linde to address several outstanding problems in cosmology, such as the horizon problem and the flatness problem.
The horizon problem arises from the fact that regions of the universe that are widely separated today were not in causal contact in the early universe. Yet, the observed universe appears to be remarkably homogeneous and isotropic, with a consistent temperature across large scales.
Inflation solves this problem by postulating that these distant regions were once close enough to equilibrate their temperatures before rapidly separating due to inflation.
The flatness problem concerns the observed nearly flat geometry of the universe. Inflation predicts that the expansion during its early stages would have flattened the curvature of space, explaining why our universe appears flat today.
During the inflationary epoch, the universe was dominated by a hypothetical field called the inflaton. This field had unusual properties, causing a repulsive gravitational effect that drove the exponential expansion. The rapid expansion stretched quantum fluctuations in the fabric of space, amplifying them and laying the seeds for the formation of large-scale structures like galaxies and galaxy clusters.
After a brief period of inflation, the inflaton field decayed, releasing its energy into particles and radiation, which eventually led to the hot, dense state of the early universe described by the Big Bang theory. The predictions of cosmic inflation have been confirmed by various observations, including the cosmic microwave background radiation and the distribution of galaxies.
In addition to resolving the horizon and flatness problems, cosmic inflation provides a framework for understanding the origin of the structures observed in the universe and the distribution of matter on large scales. It also offers a potential explanation for the origin of the density fluctuations that ultimately led to the formation of galaxies and other cosmic structures.
While the exact details of inflation and the underlying physics of the inflation field remain topics of active research, cosmic inflation is widely accepted as a crucial component of our current understanding of the early universe. It has become an integral part of the inflationary Big Bang model, providing a plausible explanation for the observed features of our universe.
8. Time Dilation
According to Einstein’s theory of relativity, time is not constant but can be influenced by gravity and velocity. This means that time can pass at different rates depending on the strength of gravity or the speed of an object.
Time dilation is a fascinating phenomenon in physics that occurs due to differences in relative motion or gravitational fields. It refers to the slowing down or speeding up of time as experienced by observers in different frames of reference.
According to the theory of relativity, time is not absolute but rather dependent on the relative motion between observers and the strength of the gravitational fields they are in.
There are two main types of time dilation:
Special Relativity Time Dilation
Special relativity states that time dilation occurs when objects or observers are moving relative to each other at significant velocities. As an object approaches the speed of light, time appears to slow down for that object relative to a stationary observer. This effect is known as time dilation. It means that time passes more slowly for a moving object compared to an object at rest. This phenomenon has been experimentally verified using highly accurate atomic clocks in fast-moving vehicles, such as airplanes and satellites.
Gravitational Time Dilation
According to general relativity, time dilation also occurs in the presence of gravitational fields. Gravity warps the fabric of spacetime, causing time to pass more slowly in regions of stronger gravity. This means that clocks closer to massive objects, such as planets or black holes, tick more slowly compared to clocks farther away.
This effect has been observed and measured, for example, with atomic clocks placed at different altitudes or in orbit around the Earth.
Both special and general relativity predictions of time dilation have been confirmed through various experiments and observations, including the famous Hafele-Keating experiment and the precise measurements of GPS satellites.
Time dilation is a crucial aspect of the theory of relativity and has practical implications. For instance, it affects the accuracy of satellite-based navigation systems like GPS, which rely on precise timekeeping. It also plays a role in understanding the behavior of objects near the event horizons of black holes, where extreme gravitational forces cause significant time dilation effects.
Overall, time dilation is a remarkable consequence of the theory of relativity that challenges our intuitive understanding of time. It demonstrates the intricate relationship between time, space, motion, and gravity and has profound implications for our understanding of the universe.
9. Neutron Stars
Neutron stars are incredibly dense objects formed from the remnants of massive stars after a supernova explosion. They are so compact that a teaspoonful of neutron star material would weigh billions of tons on Earth.
Neutron stars are incredibly dense and compact stellar remnants that form after the core collapse of massive stars during a supernova explosion. They are one of the densest known objects in the universe, packing an immense amount of mass into a relatively small volume.
Here are some key features of neutron stars:
Composition and Density: Neutron stars are primarily composed of tightly packed neutrons, hence the name. They are so dense that the matter within them is in a state known as neutron-degenerate matter. The density is so extreme that a teaspoon of neutron star material would weigh billions of tons on Earth.
Size and Mass: Neutron stars typically have a diameter of about 10-20 kilometers (6-12 miles), making them quite compact compared to their mass. Their mass can range from about 1.4 times the mass of the Sun up to around 3 times the solar mass or more.
Strong Gravity and Escape Velocity: Due to their mass and compactness, neutron stars have a powerful gravitational pull. The escape velocity from their surface is a significant fraction of the speed of light, making it extremely challenging for anything, including light, to escape their gravitational grasp.
Rotation and Pulsars: Neutron stars often exhibit rapid rotation and emit beams of electromagnetic radiation that can be observed as pulsating signals. These objects are known as pulsars. The rotation is a result of the conservation of angular momentum during the star’s collapse. As the star rotates, the emission of radiation is observed as regular pulses, like a cosmic lighthouse.
Magnetic Fields: Neutron stars possess extremely strong magnetic fields, typically billions of times stronger than Earth’s magnetic field. These intense magnetic fields play a crucial role in the behavior and emission properties of neutron stars.
Neutron Star Types: Neutron stars can have different types depending on their characteristics. Some examples include pulsars, magnetars (neutron stars with exceptionally strong magnetic fields), and neutron stars in binary systems, which orbit another star and can accrete material from it.
Neutron stars are fascinating objects that provide valuable insights into the physics of extreme conditions, gravity, and matter under extreme pressures. Their study contributes to our understanding of stellar evolution, supernova explosions, and the behavior of matter in the most extreme environments in the universe.
10. Possibility of Extraterrestrial Life
With billions of galaxies and countless stars in the universe, the existence of extraterrestrial life remains a tantalizing possibility. Scientists continue to search for signs of life beyond Earth through initiatives such as the search for exoplanets and the exploration of potential habitable environments.
The possibility of extraterrestrial life is a captivating and open question in the field of astrobiology. While no direct evidence of extraterrestrial life has been found to date, the vastness of the universe and the existence of potentially habitable environments suggest that life beyond Earth may be plausible.
Here are some key factors and considerations regarding the possibility of extraterrestrial life:
Abundance of Planets
The discovery of numerous exoplanets (planets outside our solar system) in the habitable zone of their host stars has increased the likelihood of finding environments conducive to life. Estimates suggest that there could be billions of potentially habitable planets in our galaxy alone.
Existence of Liquid Water
Water is a crucial ingredient for life as we know it. The presence of liquid water, either on the surface or within subsurface oceans, is considered a vital factor in the search for habitable environments. Enceladus and Europa, moons of Saturn and Jupiter, respectively, are among the intriguing candidates with subsurface oceans.
Extremophiles on Earth
The discovery of extremophiles—microorganisms thriving in extreme conditions on Earth—has expanded our understanding of the potential range of habitable environments. Life forms that can survive in extreme temperatures, high pressures, or acidic conditions indicate that life may have adapted to diverse and challenging environments elsewhere in the universe.
Organic Molecules and Building Blocks of Life
Organic molecules, including amino acids and complex organic compounds, have been detected in various environments, such as comets, meteorites, and the atmospheres of other planets. These discoveries suggest that the building blocks of life are widespread in the cosmos.
Potential for Life Beyond Earth
While Earth-like life is often a focus of astrobiology, the concept of “life as we don’t know it” acknowledges the possibility of forms of life-based on different biochemistries and alternative solvents. This widens the range of potential habitats and organisms that could exist.
Technological Signatures
The search for extraterrestrial intelligence (SETI) explores the possibility of detecting signals or signs of advanced civilizations. Radio signals, techno-signatures, or other forms of communication could provide evidence of intelligent life beyond our planet.
While the potential for extraterrestrial life exists, it is important to approach the topic with scientific rigor and remain open to the possibility of both simple and complex forms of life. Ongoing and future missions, such as the exploration of Mars and the study of exoplanets, will continue to contribute to our understanding of the potential for life beyond Earth.
You may also like:
- 10 Amazing Facts About the Universe
- 10 Interesting Facts About the Bermuda Triangle
- 15 Interesting Facts About Human Body
- 20 Great Indian Discoveries & Inventions
Conclusion
These mind-blowing facts about space and universe illustrate the vastness, complexity, and wonders of the cosmos. They inspire awe and encourage further exploration and scientific inquiry into the mysteries that surround us.